Main results of the experimental and theoretical research completed in 2009
|
|
Constraining the Dark Energy equation of state parameter with observations of structure growth
In 2009, the international team headed by the scientists from IKI have published the results of studies of Dark Energy using observations of the growth of large scale structures in the Universe. This study presents the first firm evidence that Dark Energy stiffles the growth of massive clusters of galaxies over the last several billion years. The observations of suppressed growth of structures is a new evidence for existense of Dark Energy, and they also allow us to improve significantly (by a factor of ~2) the constraints on its empirical properties. Combination of the cosmological measurements using growth of galaxy clusters, cosmic microwave background, and distances to the type Ia supernovae now constrains the equation of state parameter to +-5%, and its value, w0=-0.99+-0.045, is in agreement with the value expected for the Einstein's cosmological constant (w0=-1). The observational determinations of the Dark Energy properties thus continue to converge on the boundary which separates "gentle" acceleration from the so called Big Rip (expected for w0<-1) when the dark energy density increases as the Universe expands, whcih in the far future would result in destruction of all gravitationally bound structures.
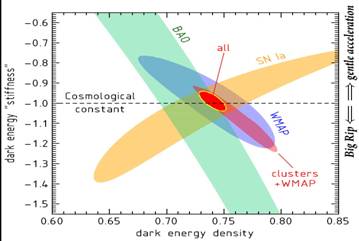 | Figure caption:
Constraints on the dark energy density and equation of state parameter, w. Different colours show the constraints from individual
cosmological datasets, and the small red ellipse in the center illustrates the combined constraints. The dashed line corresponds
to the Einstein's cosmological constant.
|
Authors: A.V. Vikhlinin, R.A. Burenin, A.A. Voevodkin, M.N. Pavlinsky
Direct Measurement of the Contribution of Point Sources to the Galactic Ridge X-ray Emission in Record Deep Observations by the Chandra Observatory
The existence of the enigmatic X-ray emission extended along the plane of our Galaxy, and also discovered in other galaxies,
puzzled the astrophysicists around the world for more than 25 years. Due to research carried out in our group in 2006-2008,
a solution of this problem emerged. To verify our hypothesis that the Galactic Ridge X-ray Emission is the cumulative emission of
a great number of weak X-ray sources, a key experiment was needed, namely ultradeep (with a flux limit of 1å-17 erg/s/sq.cm) observations
of a region of the Galactic ridge with an instrument having an angular resolution of at least 1-2 arcseconds. In 2008, such observations,
with a total exposure of 0.9 million seconds, were performed by the Chandra orbital observatory following our proposal.
Our analysis of all the available observations (nearly 1 million seconds) of this field has confirmed the
estimates we did previously based on indirect arguments and has led us to the following conclusions:
- In a typical Galactic Ridge region, a large number of faint X-ray sources is detected, with a column density of 100 thousand sources per square degree,
- 88 +/-12 % of the Galactic Ridge X-ray Emission in the energy range 6-7 keV is resolved into discrete point sources,
- Ihe integrated sources counts as a function of flux is in good agreement with the numbers of sources expected from the statistics of X-ray sources in the solar neighborhood. These results are published in the Nature journal.
Revnivtsev M., Sazonov S., Churazov E., Forman W., Vikhlinin A., Sunyaev R., 2009, Naturå, 458, 1142
Fig. Image of the Galactic Ridge - X-ray emission extended along the Galactic disk. The inset shows an image of the small field near the Galactic Center that was observed by the Chandra X-ray Observatory for a million seconds. The huge density of X-ray sources (at the achieved sensitivity, ~100 000 objects per square degree) is apparent.
Authors: M.G. Revnivtsev, S.Yu. Sazonov, E.M. Churazov, A.V. Vikhlinin, R.A. Sunyaev
Experimental detection of new phenomenon: non-adiabatic resonant acceleration of ions at the region of closed magnetic field lines in the distant parts of Current Sheet of the Earth’s magnetotail.
It was traditionally suggested earlier by the majority of researchers that an acceleration of charged particles in the Earth magnetotail occurs near a magnetic reconnection region. On the basis of detail analysis of ion and electron velocity distribution functions registered in more than 1000 crossings of the Plasma Sheet Boundary Layer (PSBL) by Geotail and Cluster spacecrafts, we revealed that during quiet or moderately disturbed geomagnetic periods ions are non-adiabatically accelerated in the distant Current Sheet (CS) at the region of closed magnetic field lines, i.e. earthward and rather far from the distant magnetic X-line. This acceleration has quasi-steady character and occurs at several localized acceleration sources (resonances) operating simultaneously (Fig.1). Accelerated ions are ejected to the PSBL, where they form field-aligned ion beams (beamlets), which are localized both in velocity and physical space. Duration of such beams may exceed 20 min (Fig.2). In the regions between resonances ions experience strong scattering and are trapped within the CS.
So that, during quiet periods the distant part of the CS resembles diffractive lattice: localized sites of reflection (i.e. directed acceleration) intermit with dark spots of strong ion scattering and trapping. Instead of acceleration in the one large-scale source, which usually forms near the reconnection region, ions are accelerated simultaneously at several localized sites, located at the region with finite and positive Bz. Energy collimated ion beams are ejected to the PSBL and form its discrete structure which is often observed in the magnetotail.
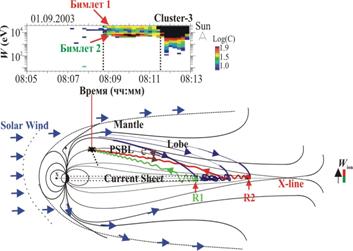 | 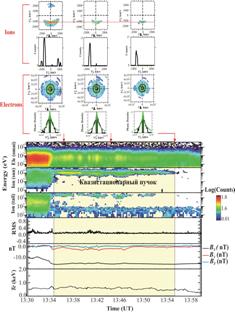 |
Fig.1. Simultaneous observation of two beamlet with essentially different energies which were accelerated in the CS at different resonant sources R1 and R2.
| Fig.2. Geotail observation of quasi-steady energy collimated ion beamlet which was accelerated in the distant CS at the region of closed magnetic field lines.
|
- Grigorenko, E. E., M. Hoshino, M. Hirai, T. Mukai, and L. M. Zelenyi, J. Geophys. Res., 114, A03203, doi:10.1029/2008JA013811, 2009.
- Grigorenko E.E., L.M. Zelenyi, M.S. Dolgonosov, J.-A. Sauvaud, Spatial and temporal structures in the vicinity of the Earth’s tail magnetic separatrix. Cluster observations, Book of Proceedings of 15th Cluster Workshop and CAA School, p.p. 435-453, 2009.
- E. Grogorenko, R. Koleva. Variability of discrete plasma structures in the lobe-plasma sheet interface, Compt. Rend. Acad. Bulg. Sci. v.62, No11, p. 1449-1456,
2009.
Investigation of processes of geomagnetic storm generation by various types of solar wind streams
Numerous experimental data testify that the geomagnetic storms, generated by the different
interplanetary phenomena, manifest themselves in different development of disturbances inside magnetosphere. On the basis of the OMNI archive data on interplanetary conditions during 1976-2000 we made statistical analysis of interplanetary drivers of 798 geomagnetic storms with Dst < -50 nT. We categorized various large-scale types of solar wind as interplanetary drivers of storms: corotating interaction region (CIR, 145 storms), Sheath (96), interplanetary CME (ICME) including magnetic cloud (MC, 62) and Ejecta (161), and drivers for 334 storms (”Inde-
terminate” type) have not been determined because of data gaps for numerous intervals of solar wind. To make the given analysis,
we used for the first time a method of double superposed epoch analysis which uses two reference times: onset of magnetic storm and
the minimum Dst index (see Figure). On the one hand, by means of this method the earlier received results have been confirmed,
and on the other hand, new results are received:
- For the first time clear indications that there are distinctions in efficiency
of two subclasses interplanetary ÑÌÅ (MC and Ejecta) and also Sheath before them have been received (see figure)
- The fact that there is «a memory of prehistory» for process of generation of a magnetic storm has been confirmed by independent method
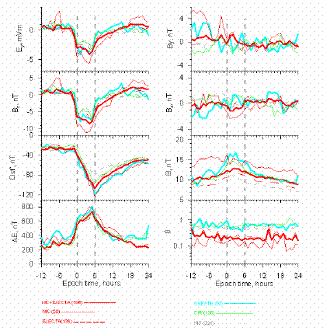 | Figure 1. Temporal variations of electric field Ey, Bz component of interplanetary magnetic field (IMF), magnetospheric Dst* and AE indexes(left ), B, Bx components and magnitude of IMF, ratio of thermal to magnetic pressures (plasma beta-parameters) for different interplanetary drivers(see designation under figure).
Durations of main phase of all storms have been re-scaled in a such manner that, respectively, onsets (left dashed line, time”0”) and minima of Dst index (right dashed line, time “6”) for all storms coincide.
|
Yermolaev Yu.I., I.G. Lodkina, N.S. Nikolaeva, M.Yu. Yermolaev, Statistic research of effect of interplanetary conditions on geomagnetic storms, Cosmic Research, 2010, (in press)
Yermolaev Y.I., N.S. Nikolaeva, I.G. Lodkina, M.Yu. Yermolaev, Specific interplanetary conditions for CIR-, Sheath-, and ICME-induced geomagnetic storms obtained by double superposed epoch analysis, Adv.Space Res., 2010 (in press, http://arxiv.org/PS_cache/arxiv/pdf/0911/0911.3315v1.pdf)
Yuri Ivanovich Yermolaev, Head of lab, Space Research Institute, Russian Academy Sciences, tel. +7-495-333-1388, email yermol@iki.rssi.ru
Particle acceleration and heating of solar flare plasma heating
For the first time a proportionality of the flare plasma temperature to the logarithm of hard X-ray intensity was discovered
during pre-flare and impulsive phases of the 2006 December 6 solar flare. The hard X-ray intensity was estimated from count rate
of the anticoincidence system of the spectrometer on INTEGRAL (ACS SPI) with energy threshold of 80 keV for gamma-quanta.
Moreover non-thermal processes were observed about 5 min earlier than the onset of thermal emission. These demonstrate that electrons generating hard X-ray intensity have been an initiator and main source of flare plasma heating, the particle acceleration and the plasma heating is a system with positive feed back. The proportionality disappears after beginning of plasma expansion, when plasma cooling becomes more effective than its heating by non-thermal electrons. These effects were not observed earlier due to low sensitivity of detectors (for example, NASA RHESSI).
Preliminary analysis of some other events confirms our findings.
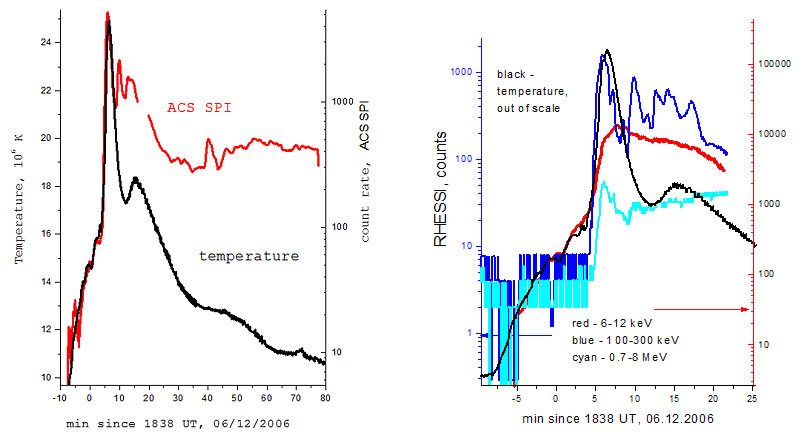
Fig. 1. Left panel: comparing of the flare plasma temperature with the logarithm of hard X-ray intensity (>80 keV, ACS SPI).
Right panel: comparing of the flare plasma temperature with the logarithm of RHESSI count rates in different X-ray energy ranges.
- A. Struminsky and I. Zimovets, OBSERVATIONS OF THE 2006 DECEMBER 6 SOLAR FLARE: ELECTRON ACCELERATION AND PLASMA HEATING, Astronomy Letters, in press 2010.
- A. Struminsky: ‘Relationship between plasma temperature and HXR intensity from INTEGRAL’, WorkGroup 2, 9th RHESSI workshop, September 2009, Genova, Italy
- A. Struminski: ‘Cross calibration with Anti-Coincidence System of Spectrometer on INTEGRAL (ACS SPI)’, WorkGroup 3, 9th RHESSI workshop, September 2009, Genova, Italy
- A. Struminsky and I. Zimovets, PARTICLE ACCELERATION AND HEATING OF SOLAR FLARE PLASMA, The Sun: from active to quite, International Coronal Workshop, 19-23 October, 2009, FIAN, Moscow, Russia.
Authors: A.B. Struminsky and I.V. Zimovets
|
|